BMB 496: Independent Studies
As a university, Penn State has a vigorous and extensive research enterprise. Faculty are awarded grants by government and private agencies to conduct research in the many academic disciplines within the university structure. Undergraduates who qualify are invited to participate in the on-going research programs of BMB faculty. Typically, between 70 and 80 students are engaged in undergraduate research during the Fall and Spring semesters. Most of these students are juniors and seniors. Participation in undergraduate research requires that an application be submitted which is reviewed by faculty members selected by the student.
Applications must be submitted by the 8th week of classes for 496 positions to be filled the following semester. The deadline for admission to summer and fall semester positions will be the eighth week of the preceding Spring semester.
Application Deadlines:
- Applications for Summer/Fall 2025 are now being accepted.
- The Summer/Fall 2025 application cycle is open from Monday, February 3, 2025 - Monday, March 3, 2025.
- The deadline to apply is Monday, March 3, 2025.
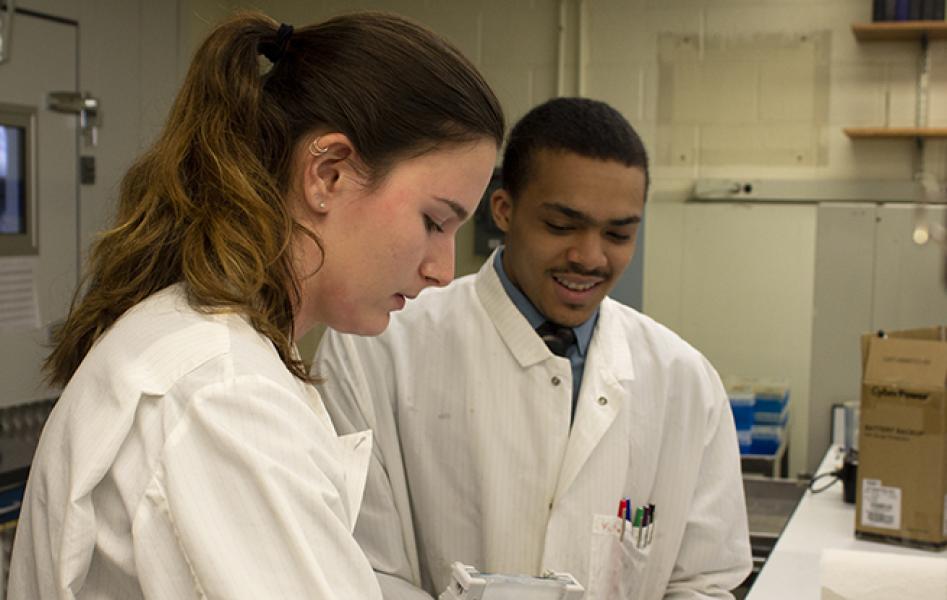
Steps to Apply
Step 1: Review guidelines for arranging an independent research position.
Step 2: Review the faculty research interests and student selection criteria list below.
Step 3: Select four faculty laboratories with whom you would be most interested to work with and indicate them on the application.
BMB 496 Research Interests and Student Criteria
The genome in eukaryotes is organized into chromatin, a condensed superstructure that evolved to keep DNA compressed to fit inside the nucleus, to maintain a tight control over the gene expression and to minimize potential damage. To maintain and adjust the genome, cells use numerous regulators; Some of those regulators rely on covalent modifications of the "packaging" histone proteins, others use the energy in the form of ATP to perform their functions. The latter are called" ATP-dependent chromatin remodelers" and are responsible for regulating DNA accessibility that is critical in processes such as DNA repair, gene expression or silencing.
The ATP-dependent chromatin remodelers are macromolecular machines that can be classified in four main families that share a common ATPase core, but contain additional domains that affect their functionalities, behavior or time at which they are activated. These complexes can contain just a single ATPase or can employ additional accessory subunits that change their targeting and behavior.
In the Armache lab, we are interested in structural and mechanistic studies of ATP-dependent chromatin remodeling complexes that are important during the DNA-damage repair and whose mutations are associated with human diseases (cancer). We probe the structures using a range of biochemical and biophysical approaches, but the main technique of the lab is called "cryo-electron microscopy" (cryo-EM). In this technique, powerful microscopes shoot electrons into a deeply frozen sample, producing images that are beyond the capabilities of optical microscopy. This technique has been responsible for some of the most exciting discoveries in recent years, including the visualization of the SARS-CoV-2 viruses.
SELECTION CRITERIA:
Highly motivated, detail-oriented, hard-working students, enthusiastic about research; commitment of 10 hours per week for at least three semesters.
Transcription by RNA polymerase (RNAP) is regulated at the level of initiation, elongation and termination. Our lab is interested in elucidating the regulatory mechanisms that control gene expression after transcription initiates. The mechanisms that we study involve regulated transcription elongation, transcription termination, translation initiation and mRNA stability.
We are investigating the mechanism by which two general transcription elongation factors (NusA and NusG) regulate RNAP pausing during elongation and the mechanism of NusA-dependent termination.
CsrA-mediated global control is another major project in the lab. CsrA is an RNA binding protein that regulates several hundred genes by repressing translation initiation and/or by affecting the stability of target mRNAs. CsrA activity, in turn, is controlled by the stress response sigma factor (RpoS), two non-coding regulatory RNAs (sRNA), and by repressing its own translation. We are continuing to elucidate novel CsrA-mediated regulatory mechanisms and connections with additional stress response pathways, as well as identification of novel components of the Csr regulatory circuitry.
We use a combination of genetic, biochemical and global genomic approaches to address these regulatory mechanisms using Bacillus subtilis and Escherichia coli as model organisms.
SELECTION CRITERIA:
Students with a strong interest in science and the desire to pursue graduate research training. A commitment of 10-15 hours per week in the lab and the desire to spend the summer working in the lab.
Bai lab is interested in studying the relation between chromatin and gene expression at single cell/single molecule level by using a combination of biophysical, biochemical, genetics, and computational methods. The long term goal of our lab is to identify sequence and chromatin features that affect the level, noise and dynamics of gene expression, to understand how these chromatin features are established and characterize their cell-to-cell variability and dynamic change, and finally, to explore how these molecular processes affect cell phenotype.
SELECTION CRITERIA:
Candidates should have some basic knowledge / training in molecular biology and genetics, and are interested in quantitative biological problems.
RNA serves important roles in biology. Our lab works on mechanistic enzymology of ribozymes using chemical and biochemical approaches. We also study how RNA folds in vivo both in an organism and in complex solutions mimicking the cytoplasm. Work in general is at the interface of chemistry and biology. Students will have their own projects and use methods ranging from thermodynamics and kinetics to molecular biology and bioinformatics.
SELECTION CRITERIA:
Strong interest in chemistry, bioinformatics, or molecular biology. Commitment to at least two years in the lab, preferable with one summer. Willingness to write a thesis either through SHC or SCIRES. See our website on undergraduate research for more details.
The human microbiome may be considered a “hidden organ”; however, while all of our hearts have the same structure and function, we each have our own unique microbiome that can change in as little as a day. Our lab investigates how microbes, drugs, and diet interact to shape host physiology. We use a combination of anaerobic microbiology, molecular biology, cell culture, animal studies, high throughput nucleic acid sequencing, and bioinformatics to map these interactions.
SELECTION CRITERIA:
Must be willing to commit to at least 10 hours per week for 2 semesters/1 summer minimum with a minimum GPA of 3.0.
I am interested in enzymes that use complex metal ion clusters to catalyze important and difficult chemical reactions (e.g. nitrogen fixation, water oxidation, alkane functionalization). In general, Nature has evolved far more elegant and effective strategies than chemists have yet devised to carry out these difficult reactions. In most cases, Nature’s strategies depend on her ability to synthesize structurally diverse and complex clusters of metal ions for use as catalytic cofactors. Thus, a primary objective of our research is to understand the mechanisms by which Nature assembles these clusters into the proteins in which they ultimately function as catalytic centers.
SELECTION CRITERIA:
Strong background and interest in chemistry and physics.
The Feric lab studies the fundamental principles underlying cellular organization and how anomalies contribute to age-related diseases. In particular, we investigate how phenomena occurring on different length scales–molecular, organellar, and cellular–are interconnected with each other and are maintained over time, primarily in the context of aging. To do so, we take an interdisiplinary approach using biomolecular phase transitions as the working framework. With advanced, super-resolution light microscopy techniques and quantitative analysis, we will probe the organization and function of biomolecules in droplet-like structures called condensates, primarily focusing on those found in an organelle highly implicated in aging: the mitochondrion. The simplicity of mitochondrial biology allows us to reconstitute fundamental processes, such as transcription and replication, allowing us to interrogate, both in vitro and in vivo, structure-function relationships that emerge within condensates. By identifying the mechanisms by which the cell maintains the organization and activity of its condensates over time, our work will shed light on new approaches to improve human health span and treat age-related diseases.
Selection criteria:
Highly motivated and enthusiastic freshmen, sophomores, or juniors with a >3.0 science GPA. Commitment of 10 hours/week for two or more semesters and preferably with one summer.
Cellulose, composed of β-1, 4 linked glucan chains, is the most abundant biopolymer on earth. Cellulosic biomass is an abundant renewable resource that has been recently targeted for the production of alternative transportation fuels. However, little is known how cellulose is produced and how the biopolymer is assembled. My main objective is to characterize the molecular and cellular mechanisms controlling cellulose biosynthesis in higher plants. My lab uses live cell imaging to visualize the cellulose synthesis machinery in living plant cells. Through molecular, biochemical, and genetics approaches, we identified several proteins that could potentially involve in the assembly, delivery, and regulation of the cellulose synthesis machinery. We aim to unravel the mystery of cellulose biosynthesis as technology, genetic and genomic tools are well developed, particularly in Arabidopsis. Considering cellulosic biomass are expected to become one of the main sources of biomass for the production of renewable biofuels in the near future, the data generated through our research may be of great interest for energy producing agents.
SELECTION CRITERIA:
Highly motivated freshman, sophomores, and juniors who are willing to commit 10 hours per week for at least 2 semesters.
Inborn errors of metabolism are congenital birth defects caused by mutations in metabolic enzymes. In the Hanna-Rose lab we use the nematode C. elegans to model such diseases and to decipher the molecular mechanisms that result in phenotypic outcomes involving both behavior and development. Specifically, we study purine and NAD+ metabolism. Perturbation to either of these metabolic processes can have surprisingly organ or tissue-specific phenotypes as well as phenotypes more subtle or nuanced than expected based on the core metabolic role of the metabolites being produced. For example, purine biosynthesis defects in humans cause muscle dysfunction and fascinating neurological phenotypes including autistic-like and compulsive behaviors. We can recapitulate similar phenotypes in C. elegans where we combine traditional and reverse genetics techniques with modern metabolomics analysis to unravel the complex roles of the metabolic pathways and their constituent metabolites in both normal physiology and disease pathology.
SELECTION CRITERIA:
Enthusiastic, highly motivated, hard-working student; commitment of 10 hours per week for several semesters; first and second year students preferred; no seniors.
Virion Assembly and Transmission Mechanisms of Zoonotic RNA Viruses
Jose lab is interested in understanding the pathogenesis of arthropod-borne alphaviruses and flaviviruses such as Zika, West Nile, dengue, Powassan, and chikungunya virus. Diseases caused by these viruses remain an international public health concern as there are no effective prophylactic treatments or vaccines against most of these human pathogens. During replication, these positive-strand RNA viruses modify the host cell to induce virus replication structures that help evade the innate immune system. These viruses cause arthralgia, microcephaly, encephalitis, and fatal hemorrhagic fever. We investigate the virus-host and virus-vector interactions involved in virus entry modification of the host system for virus replication and dissemination using various tools in virology, molecular biology, mass spectrometry, EM, and live cell imaging. We aim to understand the mechanisms of virus-host interactions and to perturb them to develop new strategies to control and combat these pathogens.
Projects currently available for undergraduate research include the following:
- Mechanisms of tunneling nanotube formation by ZIKV and virus-host interactions at the maternal-fetal interface: Zika virus (ZIKV) has the unique ability of human-to-human transmission vertically from mother to fetus and horizontally through sexual contact. Vertical transmission is of particular concern because it can result in severe fetal microcephaly. However, scientists have struggled to understand the mechanisms that underlie maternal-fetal transmission of ZIKV on a cellular and molecular level. We investigate this phenomenon by studying host cell interactions and structure manipulations in ZIKV-infected cells.
Research questions:
- What are the roles of ZIKV nonstructural proteins in inducing modified cell structures?
- What mechanisms are involved in hijacking the host pathways for intracellular modification, facilitating virus replication and dissemination?
- What pathways in the placenta are essential for ZIKV infection?
- Are there significant interactions between ZIKV and specific organelles, and how do they affect cell metabolism and viral transmission?
- Defining the molecular interactions required for flavivirus genome packaging and virus assembly: Despite decades of research, many flavivirus assembly and replication aspects remain elusive. We investigate the intricate mechanisms underlying flavivirus assembly by defining the critical roles of structural and nonstructural proteins in viral genomic RNA packaging. A mechanistic understanding of the essential interactions in genome packaging and virus assembly will lead to identifying novel targets and strategies to combat these vector-borne pathogens.
Research questions:
- How do intracellular dynamics of viral proteins and RNA coordinate the assembly of flaviviruses?
- What host pathways are involved in virus production and cellular membrane modification?
- Identifying the entry, replication, and vector specificity determinants of tick-borne flaviviruses: Penn State’s Eva. J Pell Lab facilities to investigate the molecular mechanisms in virus replication, assembly, and neurotropism of BSL-3 flaviviruses, including the tick-borne Powassan virus. We study the emergence and genomic variability in the transmission dynamics of the Lineage II Powassan virus, also known as deer tick virus, which has become a public health concern in recent years due to its prevalence in environmental tick samples.
Research questions:
- What are the molecular drivers that are contributing to the emergence of tick-borne flaviviruses?
- Are genome changes in circulating Powassan viruses contributing to increased human infection and disease incidence?
- What are the virus-host interactions acting as the bottlenecks of tropism, and how are these changing over time?
- How do neurotropic flaviviruses, including Powassan virus, cross the blood-brain barrier, causing encephalitic disease?
- Roles of alphavirus proteins 6K, E1/E2, and nsP1 in virus replication, assembly, and transmission. Mosquito-borne alphaviruses such as chikungunya, Mayaro, and Eastern equine encephalitis viruses cause high morbidity and mortality in their mammalian hosts. Intracellular mechanisms that drive alphavirus assembly and budding represent promising antiviral targets. One potential drug target is the alphavirus-encoded ion channel protein 6K, which plays an essential part in virus budding by enabling the formation of cytopathic vacuoles-II and envelope spike protein transport to the plasma membrane.
Research questions:
- Functional role of 6K ion channel in alphavirus glycoprotein trafficking and CPV-II formation
- Mechanism of nsP1 in modifying the cytoskeleton and mediating the replication complex transport
- How do CHIKV E1/E2 envelope protein variants, individually and in assemblages, affect vector competence in Ae. aegypti and Ae. albopictus?
SELECTION CRITERIA:
Highly motivated sophomores and juniors. GPA above 3.3, Preferred majors: BMB, MICRB. Undergraduates are required to spend (10-15 hours/week) in the lab, for 2 or more semesters and for 1 or more summers.
The lab is interested in a self/non-self recognition mechanism in flowering plants termed self-incompatibility, which allows the female reproductive organ, the pistil, to distinguish between self and non-self pollen. Self-pollen is rejected whereas non-self pollen is accepted for fertilization. Thus, self-incompatibility prevents inbreeding and promotes outcrosses, and is beneficial to plants. Our ultimate goal is to use molecular genetic and functional genomic approaches to identify all the genes involved in the recognition and rejection of self-pollen by the pistil, and decipher the biochemical mechanism of this process. Petunia inflata, a wild relative of garden petunia, is used in all the studies.
SELECTION CRITERIA:
Minimum GPA - 3.00; highly motivated freshmen, sophomores, and juniors preferred; minimum commitment of 2 academic semesters plus one summer.
We are interested in learning the atomic-resolution details of the spatial organization of highly structured RNA molecules and complexes of such molecules with RNA-binding proteins. Using cryo-electron microscopy and X-ray crystallography we can determine three-dimensional structures of molecules to the finest details; using a combination of structural and biochemical studies we can answer a very broad variety of fundamental questions, ranging from the mechanisms of substrate recognition and catalysis, to the structural and functional roles of individual parts of a molecule or complex.
SELECTION CRITERIA:
High motivation; commitment to spend at least 5 hours per week for at least 3 semesters; a decent science GPA.
We study inherited diseases caused by expansion of trinucleotide repeats (repeated stretches of GGC, GAA, CAG) at specific positions in the genome. Those expansions cause severe neurological disorders, which worsen with age and finally lead to disability and premature death. The mechanisms of trinucleotide diseases still remain a mystery and there is currently no cure. To understand the mechanisms of those diseases we monitor how transcription and replication are going through those repeats in the model systems, including mammalian cultured cells. We use cloning, two-dimensional electrophoresis, southern and northern hybridization.
SELECTION CRITERIA:
Highly motivated students. Minimum GPA 3.0. Willing to spend in the lab 5-10 hours a week.
The importance of iron as an essential element is underscored by the fact that iron-containing proteins play a pivotal role in almost every aspect of life. Our laboratory uses spectroscopic techniques, such as Mössbauer, EPR, and UV/VIS, to obtain detailed information about the nature of the Fe-sites in these proteins. When these spectroscopic methods are combined with kinetic techniques (rapid freeze-quench, stopped flow), it is possible to monitor the changes of the iron during a reaction and consequently, these studies allow us to determine the reaction mechanism of such enzymes.
SELECTION CRITERIA:
Strong background and interest in chemistry and physics required. Must be sufficiently motivated to work 10 or more hours for 3 or more semesters. Honors program preferred.
Research focus: Understanding cell signaling mechanisms that control tissue growth and organ development through molecular, genetic, and bioinformatics approaches.
SELECTION CRITERIA:
Some background in bioinformatics, genetics, and molecular biology. Strong interest in understanding developmental mechanisms and their relevance to human diseases. Sophomore or junior. Two to four semesters.
To understand the mechanisms and pathways of protein evolution, we take advantage of the large amount of amino-acid sequence and protein-structural information available in databases. Projects appropriate for students would include analyzing sequence-structure relationships in families of proteins. The work would involve learning how to run standard bioinformatics software, and writing simple programs.
Selection criteria:
A curiosity about protein-structure, and facility with using computers, including some programming skills.
Our lab aims to understand how regulatory proteins such as transcription factors find their targets in the genome. We develop machine-learning algorithms to analyze large collections of experimental data from high-throughput sequencing assays such as ChIP-seq, ChIP-exo, RNA-seq, and DNase-seq. We also apply these computational methods to understand how transcription factors bring about changes in cell identity during development and cellular programming. We need motivated undergraduates to help us develop tools for analyzing and visualizing large collections of genomics datasets.
SELECTION CRITERIA:
Highly motivated freshmen, sophomores, or juniors preferred. Prior experience with computer programming languages required.
The McReynolds Lab is broadly interested in understanding the biochemistry behind aging, and its intersection with stress, with the long-term goal of identifying strategies that promote healthier aging. Characterizing metabolic adaptation to acute and chronic stress in aged-related disease models will help illuminate age-related metabolic defects and reveal metabolic homeostasis mechanisms. As scientists working at the interface of chemistry and biology, the lab's research program will take advantage of high-resolution liquid-chromatography mass spectrometry (HR-LCMS) coupled to in vivo isotope tracing to:
- Elucidate how NAD+ homeostasis is achieved and fails over the lifespan
- Uncover how toxic circulating small molecules contribute to aging and aged-related disorders
- Reveal stress-induced metabolic alterations in aging and aged-related disorders
SELECTION CRITERIA:
GPA requirement is flexible (~3.3 is ideal but will consider lower GPAs), BMB major interested in metabolism, preferably entering sophomore or junior year.
Our lab focuses on cell envelope biogenesis in both gram-positive (Staphylococcus aureus) and gram-negative (Pseudomonas aeruginosa) organisms, two common and problematic human pathogens. We employ various functional genomics approaches to understand how the cell assembles and maintains the cell envelope, and ultimately how these processes impact virulence. Potential projects include probing membrane lipid recycling pathways and characterizing new cell envelope related antibiotic resistance determinants. Students with interests in bacterial genetics and physiology are particularly encouraged to apply.
SELECTION CRITERIA:
Minimum GPA 3.00, 10+ hours per week with a commitment for 3+ semesters and/or summers. Sophomores and juniors preferred; highly motivated freshman will be considered. Majors: BMB or MICRB
The Miyashiro lab is interested in understanding how animals acquire and maintain different strains of bacterial symbionts. Using the symbiosis established between the Hawaiian bobtail squid and the bioluminescent bacterium Vibrio fischeri as an experimental platform to model multi-strain symbioses, students will determine how different V. fischeri strains interact with a squid host and each other. Students will gain experience in microbiology and molecular biology tools..
SELECTION CRITERIA:
A strong interest in research and excitement about science. Must be willing to commit to 10-15 hours per week for at least 4 semesters. BMB, MICRB, BIOL majors preferred. Minimum GPA: 3.3.
Transcription is the major control point of gene regulation and RNA polymerase (RNAP) is the central enzyme of transcription. The long-term goal of this laboratory is to understand the mechanism of transcription and its regulation. We are particularly interested in determining X-ray crystallographic structures of RNAPs from different kind of organisms, e.g., bacteriophage (single-subunit RNAP), bacteria and archaea (multi-subunit cellular RNAP), and influenza virus (RNA-dependent RNAP). Based on the structures, we study the detailed functions of RNAP using biochemical and biophysical methods. A strong interest in research and excitement about science.
SELECTION CRITERIA:
Must be willing to commit to 10 hours per week for at least 2 semesters. BMB, MICRB majors preferred.
My laboratory will be working on a variety of problems that can be formulated and solved within the framework of comparative genomics. Below I list some of the projects. These projects represent only a small sample of fascinating questions that can be answered by comparing genomic sequences.
Comparative approach to gene annotation. Application of comparative genomics to gene prediction is an intuitive and simple way to increase its accuracy and reliability. My lab will be pursuing the following directions:
- Establish an alternative estimate of protein-coding capacity of the human genome using a comparative approach to challenge the current estimate of gene number in the human genome;
- Develop a comparative approach for the assembly of the entire gene structure including identification of exon/intron boundaries and untranslated regions.
Annotation of promoters. It appears that the gene number does not reflect the complexity of a given organism: humans only have between 30,000 and 40,000 genes, which is only a two-fold increase over C. elegans. Thus we think that the study of regulatory regions will provide answers to many fundamental evolutionary and genetic questions. Almost all currently used promoter-prediction algorithms suffer from the problem of overprediction (very high rate of false-positives). We are going to initially approach this problem by identifying groups of conserved regions and studying their combinations.
Development of innovative web-based tools and databases.
Evo-bio project. With new genomes coming out of sequencing centers every week, comparative analysis becomes the most powerful approach to unraveling the biological meaning of genomic sequences. Comparative genomics relies heavily on algorithms developed by evolutionary biologists. However there is a problem — implementation. The idea of evo-bio project is to develop a set of PERL-modules implementing numerous sequence analysis algorithms developed by evolutionary biologists.
SELECTION CRITERIA:
GPA 3.0 or above. Willingness to learn computational techniques in biology. Willingness to commit to 5-10 hours per week for at least 2 semesters.
Proteins are regulated in many ways, including by binding of small molecules, other proteins and biological molecules. In many cases, these binding events result in structural changes across the protein that direct subsequent biological functions. Because these changes are often very subtle and can be invisible in crystal structures, it is not trivial to determine how protein motions are correlated with biological activity.
Our goal is to use MD simulations to investigate conformational dynamics in protein complexes and determine how these are altered in various functional states. We will then use complementary biochemical and structural techniques to determine molecular mechanisms of long-range communication and signaling in proteins. By revealing how different conformational states are correlated with biological outcomes, we will develop strategies to control protein function for desirable applications. Additionally, an important, more general goal of the group is to contribute to the field by determining what atomistic protein MD simulations teach us about actual biological function. We will do this by constantly connecting computational data to functional, experimental data.
SELECTION CRITERIA:
Enthusiastic, highly motivated, hard-working students; willing to commit at least 10 hours per week for 2 semesters/1 summer minimum. Preference will be given to 1st and 2nd year students. Minimum science GPA 3.0. Students interested in integrating computational simulations with experiments are encouraged to apply.
Mechanisms that regulate tissue regeneration with a focus on understanding the response to anemic and hypoxic stress.
Work in the Paulson lab focuses on the mechanisms that regulate tissue regeneration. Throughout adult life, tissues become damaged and must be repaired or regenerated to maintain homeostasis. We focus on the maintenance of erythrocytes or red blood cells. Steady state erythropoiesis generates new erythrocytes at a constant rate of about 2.5 x 106/second. Despite this enormous capacity, there are times when this process cannot produce enough erythrocytes. For example, infection inhibits the ability of the bone marrow to generate erythrocytes. Similarly, in response to anemia the bone marrow production of erythrocytes is not sufficient to maintain normal blood levels. At these times stress erythropoiesis rapidly produces large numbers of erythrocytes to maintain homeostasis until the bone marrow can resume production. This process is a stem cell mediated process as hematopoietic stem cells directly generate stress erythroid progenitors. Furthermore, the signals that regulate this process are distinct from steady state erythropoiesis and are more associated with embryonic development than hematopoiesis suggesting that stress erythropoiesis is similar to other tissue regeneration processes.
SELECTION CRITERIA:
Science major, GPA 3.0 or higher. Must be able to commit 3 semesters. Possibility of summer research as well.
We are interested in determining the molecular mechanisms of toxicity of dioxin (TCDD) and other polycyclic aromatic hydrocarbons (e.g. benzo(a)pyrene). In addition, we are determining how the Ah receptor, which mediates the biological response to dioxin is regulated. The role of the Ah receptor in normal biological processes is currently our major focus. In particular, we study the role of the Ah receptor in barrier tissues (e.g. skin, gastrointestinal tract). And how this receptor could be use to enhance health and as a drug target. Yet another are area of emphasis is the ability of this receptor to modulate tumor outgrowth, survival and metastatic potential. We use a wide variety of techniques including; protein biochemistry, molecular biology, transgenic mice, cell culture, RNA seq, etc.. All techniques are used in the context of mammalian models of diseases and how the Ah receptor modulates disease progression.
SELECTION CRITERIA:
Strong interest in experimental science. Minimum commitment of 2 semesters and 10 hours per week. GPA= 3.0, sophomores, juniors or seniors. Willing to work through the summer a plus.
Our laboratory is interested in delineating biological sites of peroxisome proliferator-activated receptors (PPARs) in lipid homeostasis and cancer; the molecular regulation mediated by PPARs; and modulation of atherosclerosis and cancers through dietary or chemical activation of PPARs. Null mouse models are used extensively for these purposes. For more information about our laboratory, refer to our lab's website.
SELECTION CRITERIA:
Minimum GPA 3.00, 10+ hours per week for 4+ semesters.
Research in this laboratory is focused on the enzymology and regulation of amino acid metabolism using simple microorganisms as our model systems. The enzymes of interest are involved in the metabolism of the amino acids histidine and ergothioneine, with primary emphasis on their catalytic properties and how the formation of these enzymes is regulated. Enzymes such as histidase and urocanase are necessary for histidine breakdown but also have biological functions in higher organisms related to possible mechanisms of immunosuppression; ergothionase affects the function of ergothioneine, a compound thought to be useful for protection against oxidative damage. Projects currently available for undergraduate research include these:
- Employing an E. coli overexpression system for urocanase production, we continue to explore how its NAD+ coenzyme functions by a non-redox mechanism to permit the conversion of urocanate to imidazolone propionate. Urocanase is currently the only known enzyme in which NAD+ is employed in this manner but further understanding may suggest other enzymes which utilize a similar NAD-dependent function.
- We are examining the molecular basis for cellular toxicity exhibited by imidazolone propionate (IPA) when this compound, an intermediate in normal histidine breakdown, is allowed to accumulate due to the absence of its degrading enzyme, IPA hydrolase. Such an absence is apparently unknown in humans, suggesting that IPA hydrolase function is necessary for development, but IPA toxicity can be observed and studied in bacteria genetically lacking IPA hydrolase and this is our approach.
- Humans accumulate trans-urocanate on their skin where it can be isomerized by UV-B radiation to the cis-isomer; this cis-urocanate is known to have immunosuppresive effects possibly directed toward damage that results from excess exposure to sun (i.e. sunburn). While immunosuppresion is an interesting role for this compound, how organisms subsequently break down cis-urocanate in skin is less understood. We are studying this process in Micrococcus luteus, a common skin microbe, which may have an isomerase enzyme that catalyzes the re-formation of trans-urocanate (without sunlight) which the organism can then use as an energy source for its growth.
- Our elucidation of the function of the hutD gene product in histidine metabolism is focused on its role in regulating the activity of the Hut system repressor protein. In a variety of organisms, the hutD gene is clustered with other genes involved in histidine breakdown but its gene product's role in this process is unknown. One avenue we are exploring is that the HutD protein functions as a "helper" of the hut repressor protein to allow recognition of genomic operator sites related to histidine catabolism.
- Ergothioneine (thiolhistidine betaine) is increasingly recognized as a powerful antioxidant in tissues and thus can be of importance as a way to block oxidative and free radical damage common in all aerobic organisms. Fungi, including edible mushrooms, are a primary source of this nutritionally valuable compound. We are collaborating with others interested in studying ergothioneine production in mushrooms, but also are examining an ergothionase enzyme discovered in Agrobacterium radiobacter that is very useful as a tool for measuring ergothioneine levels. This effort includes directed mutagenesis designed to improve ergothionase catalytic properties.
SELECTION CRITERIA:
Students who are interested should have completed MICRB 202 and have completed, or be taking concurrently, BMB 401. A minimum GPA of 3.0 and a 2 or more semester commitment are sought.
Cancer results from of an accumulation of genetic and cellular damage and the ensuing uncontrolled cell proliferation. Eukaryotic cells respond to these challenges by activating DNA damage sensing, signaling and repair pathways that are stimulated by damage to DNA and other stresses. Activation of these pathways cause the execution of cell cycle delays, referred to as checkpoints, and the expression of DNA repair genes. Alterations in these functions are known to predispose people to cancer and other diseases. Dr. Reese’s laboratory uses a combination of biochemistry, genetics, genomics and molecular biology to study the mechanisms controlling stress-induced changes in gene expression in eukaryotes. The two main focus areas of the lab are the role of transcription and mRNA decay factors in regulating the DNA damage response and the control of transcription elongation by protein complexes and chromatin.
SELECTION CRITERIA:
Minimum 3.3 Science GPA; BMB, MICRB or Biology majors. Interest in a career in biomedical research. Highly motivated freshman and sophomores only. 6-10 hrs per week with at least two >3hr blocks of time.
We are interested in the subcellular organization of neurons. Neurons are specialized to integrate information and to send signals over long distances. A fundamental part of this specialization is the division into axons, dendrites and cell body. We study how these three major compartments are established and maintained using Drosophila genetics and confocal microscopy. One of the major projects is to understand how the microtubule cytoskeleton differs between axons and dendrites, and how these differences contribute to polarized trafficking of proteins in neurons. We are also studying neuronal responses to injury including regeneration.
SELECTION CRITERIA:
Undergraduates who join the lab must be highly motivated, spend at least 10 hours/ week in the lab, for 2 or more semesters, and be able to stay in the lab for 1 or more summers.
The ability of cells to move about is an important component of tissue development and injury repair. Cellular migration requires precise spatial and temporal coordination of alterations in cellular signaling and architecture. Numerous members of the Ras Superfamily of small GTPases regulate aspects of this behavior. We are studying the role of one of these GTPase families, the ADP-ribosylation factors or ARFs, in regulating motility of epithelial cells. Projects in the lab focus on the regulation and sub-cellular localization of ARF activity, and on the signal transduction cascades regulated by the ARFs. Techniques in use include mammalian cell culture, molecular biology and biochemistry.
SELECTION CRITERIA:
Enthusiastic, disciplined and self-motivated students who are interested in learning about scientific research. With a minimum GPA 3.0. Willing to commit to 10 hours/wk for at least 2 semesters.
The current view in structural biology is that the stable and unique structures adopted by proteins and nucleic acids are responsible for their functions. In this model, order is presumed to be an essential aspect of biomolecular structure. Conversely, our laboratory studies highly flexible biomolecules that do not adopt a single “most stable” structure in solution, yet these molecules retain the ability to form complexes that are critical for signal transduction and transcription. Therefore, we are motivated to ask whether a conserved functional role for disordered biomolecules represents a fundamental aspect of biomolecular structure that is currently not well addressed in biochemistry. The central theme of our research program is the development of new models for protein and RNA conformational dynamics that encompass not only well-folded systems, but lead to the application of structural biological tools to highly flexible macromolecular systems without defined structures. Our primary structural tool is NMR spectroscopy. Additionally, we seek to correlate structural information with functional outcomes through biochemical and cellular assays. To reach our goals, we study intrinsically disordered proteins involved in regulating gene expression at the level of transcription and miRNAs that regulate gene expression at the level of translation. In multicellular organisms, the task of appropriately regulating the function of each gene in the genome is dauntingly complex because both basic cellular metabolism and multicellular organization must be accounted for simultaneously. Our studies therefore address an open question in structural biology and biophysical chemistry while also providing critical insight into developmental biology and metabolism at the molecular level.
Our lab works on herpes simplex virus (HSV), a pathogen carried by most adults. The most visible infectious state of HSV occurs in skin cells, but its lifelong site of latency lies in neurons. We use a combination of neurobiology, virology, molecular biology, cell biology, bioinformatics, and genomics to understand the interactions of these viruses with neurons. Read more about our lab’s work on our website. Past undergraduate research projects have ranged from wet-bench to purely computational approaches. Currently available projects are computationally focused (bioinformatics), and include the following:
- Comparative genomics analysis of different strains of HSV (e.g. protein domain homology, phylogenetic analysis, motif searching & comparison)
- Computational prediction and comparison of regulatory domains that affect nearby coding sequences.
- Web-based tool development, for our online viral genome assembly project.
SELECTION CRITERIA:
Highly motivated sophomores and juniors with science GPA >3.3. No seniors. Preferred commitment of 2 summers, plus 5-10 hours per week in each academic semester. See our website for more information on undergraduate researchers in the lab.
Our laboratory is interested in how genes are turned off or on since such regulation is altered in cancer cells. Our goal is to determine the workings of gene regulation complexes, especially those associated with human diseases, using both biochemical and structural approaches. A focus of the lab is to determine the three-dimensional structure of gene regulation chromatin complexes by X-ray crystallography and cryoelectron microscopy. Undergraduates have also spearheaded projects to promote global science by developing inexpensive reagents such as DNA and protein electrophoresis markers (requested by more than 600 laboratories around the world). Current undergraduate projects include:
- expression and purification of cancer related proteins in E. coli,
- creating inexpensive protein molecular weight markers for size exclusion chromatography
For more information including our philosophy towards undergraduate research, please visit our website for undergraduate researchers.
SELECTION CRITERIA:
Highly motivated freshmen, sophomores or juniors in honors program preferred.
We are interested in the role of the F-actin cytoskeleton in epithelial morphogenesis – how do the cells in such tissues perform cell shape changes in such a way as to generate specific forms we see in fully developed tissues and organs? Current research focuses on the membrane associated protein Spectrin and associated proteins. We use the fruit fly Drosophila melanogaster as a powerful model system for these investigations because of the vast range of genetic, molecular and cell biological tools that this makes available to us. The specific projects available to undergraduates are always in flux, but recent examples include traditional and transgenic genetic experiments, immunofluroescent localization of proteins, live imaging of developmental processes, protein purification and calorimetry, electron microscopy and 3D reconstruction. We are also interested in the evolution of Spectrin and have projects available in this area too.
The exact techniques used will depend on the specific project picked. These could include molecular biology (Polymerase chain reaction, DNA gels and Southern blots, protein gels and Western blots, plasmid cloning and protein expression, restriction analysis etc.), cell biology (immunofluorescent analysis of protein distributions, time lapse imaging of live cells and embryos labeled with Green Fluorescent Protein (GFP), digital microscopy, etc.), protein biochemistry (expression and purification from E. coli, isothermal titration calorimetry, immunoprecipitation/ pull down experiments), genomic database searching and sequence analysis, and genetics (both classical and transgenic).
SELECTION CRITERIA:
Minimum Science GPA 3.3. Majors: BIOL, BMB, MICRB. Expectations /Requirements: highly motivated, freshmen sophomores or juniors with an interest in a long-term relationship with lab (i.e., several semesters, interest in doing summer research); 2-3 credits (10-15 hours/week). Honors thesis projects available. Biol 230 or BMB/MICRB 251/252 an advantage.
Dr. Ruobo Zhou´s research aims to develop and apply novel fluorescence imaging-based techniques to understand the compartmentalization and spatiotemporal organization of protein-protein and protein-RNA interactions that drive critical biological processes involved in basic cell functions and human diseases (e.g., neurodegenerative diseases and cancer), and to investigate the origin, structure, and function of these subcellular compartments and molecular organizations and how they collectively respond to changing conditions during growth, development, and disease at the molecular, cellular, and tissue levels. The techniques that Dr.Zhou´s lab develops and applies to study these disease-related biosystems range from single-molecule detection techniques and highly multiplexed super-resolution fluorescence nanoscopy (e.g., Stochastic Optical Reconstruction Microscopy (STORM)) to “omics” approaches such as mass spectrometry-based proteomic analysis and transcriptome-scale RNA imaging (e.g., Multiplexed Error-Robust Fluorescence In Situ Hybridization (MERFISH)). Specifically, Dr. Zhou´s research has been focused on three biosystems to illustrate the dynamics and organizations of biomolecular interactions involved in the protein and/or RNA clusters/aggregates in both normal and pathologic conditions: 1) Functional clustering of signaling proteins at the plasma membrane and membrane contact sites in neuronal cells and in T-cells; 2) Cytoplasmic signaling protein aggregation in cancer and protein/RNA aggregation in neurodegenerative diseases; 3) Mapping the genome-wide translation and RNA degradation activities in whole animal organs.
SELECTION CRITERIA:
The candidates should be highly motivated. The students who either have basic knowledge in cell/molecular biology or have some basic programming/data analysis skills are preferred.