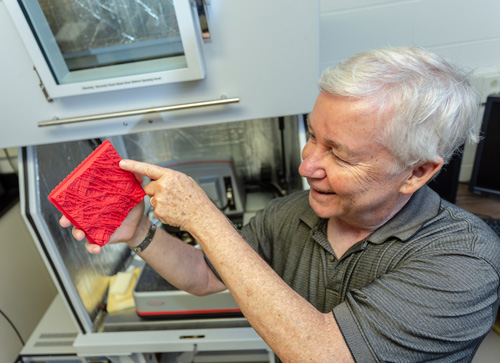
Although we don’t often think about them as such, plant cell walls are incredible structures that have an outsized impact on our daily lives.
They are amazingly and surprisingly complex—intricately arranged and many-layered lattices that can be soft or hard, flexible or rigid, absorbent or hydrophobic. They determine how every plant grows—the trailing petunia, the climbing grape, and the towering sequoia. They represent a significant portion of our diet—in fruits and vegetables, breads, pastas, and cereals. They are a critical component of biofuels and bioplastics production. And they make up many of the materials we rely on each and every day—the fibers of our clothing, the framework of our houses, the paper we write on.
“Try living without plants!” says Daniel Cosgrove. “They impact all kinds of things.”
Cosgrove directs Penn State’s Center for Lignocellulose Structure and Formation (CLSF), a multidisciplinary group of scientists with the shared goal of understanding the structure of plant cell walls and how they are made. “The whole point of the center,” he says, “is to bring together people with very different expertise to focus on these things in ways that a single lab couldn’t do.” And so, for nearly a decade, CLSF has connected experts in the biological and agricultural sciences with chemists, physicists, and engineers at Penn State and nine partnering institutions in the U.S. and U.K. to try to solve this puzzle—how lignin, cellulose, and other polymers combine to form the scaffolding upon which the entire plant kingdom is built.
“People have been using cellulose for millennia," Cosgrove says, “but yet we still don’t know its structure with respect to certain details. For instance, although we can dissolve cellulose, make it into a polymer solution, and extrude it out—which is how we get things like rayon—what you get when you do that has a very different molecular structure than what plants make. The rules and tricks plants are using to make cellulose are not very well understood, and that’s just one example; there are lots of others.”
Although many details are still unclear, scientists generally agree that plant cell walls are made up of cellulose microfibrils—tiny fibers that provide the cell wall’s tensile strength—interwoven in a matrix of pectin and other polymers. To learn more about how the cell wall is constructed, researchers at CLSF are employing a variety of experimental techniques, from quantum- and molecular-scale modeling to genetic manipulation. Cosgrove focuses primarily on high-resolution imaging, which he does with a specially adapted atomic force microscope (AFM)—a type of scanning probe microscope that measures atomic-scale interactions between its probing tip and the sample beneath.
“It’s like a record player, with a needle that rides up and down the surface,” he explains, “so you get a map of the topography and you can see the fibrillar structure. As the probe tip rides up and down the surface, at every single point it’s actually making an indentation—pushing down on the microfibril on the surface and letting up—so you can get a stiffness value and other kinds of measurements of mechanical parameters. We’ve been using the AFM to probe how the fibrils are connected to one another, their rigidity, how they’re connected to the matrix—trying to understand how these things are put together to make cell walls.”
To augment the AFM, Cosgrove has built some unique instrumentation of his own—including a device that stretches the cell wall material so he can observe what happens at the level of the individual microfibrils. “That’s a tricky business,” he says. “It sounds easy, but if your object is big and you’re measuring an area of it that’s only, let’s say, one micrometer by one micrometer, when you stretch that material the surface you were looking at has now moved into the next county, figuratively speaking. We have to go and find that piece again, the very same cell wall, and then image it and identify how the fibrils have changed. We managed to do that in a study we published last year in Nature Plants, which was the first of its kind, I believe.”
Although the AFM has been used in biology for several decades, Cosgrove is pushing its limits to see things at a finer resolution than what has been seen before. And, he points out, the instrumentation has improved significantly since he started working with it nearly 15 years ago. “Our stuff is so much better these days,” he says “and that’s because the instrument’s better and the tips are finer and also because there’s a learning curve. We’ve learned how to handle the material, and we’re still learning.”
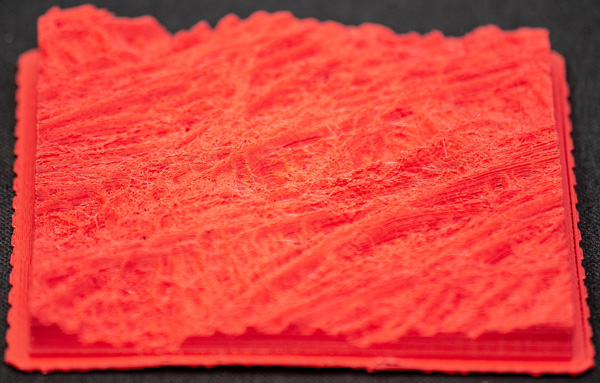
Prior to using the AFM, Cosgrove tried imaging cell walls with an electron microscope, “but at that level of resolution you have to dry the material down,” he explains, “and when you dry the material down, it changes—things collapse and shrink—and it doesn’t have the same physical properties as when it’s wet. With the AFM, you can image things while they’re wet, and that’s a prime advantage.” And like an electron microscope, the AFM is capable of nanometer- and sub-nanometer-scale resolution. “It just takes some practice to learn how to handle the materials and how to make good images,” Cosgrove says, “and that takes time.” In a recent turn of events, though, Penn State’s acquisition of a Titan Krios cryoEM has Cosgrove reconsidering electron microscopy, as a complement to his work with the AFM: to capture molecular-scale images of the enzymatic processes involved in forming cell walls. With each new development, he achieves a more-detailed view of how plants make these incredible structures.
“By exploring this,” Cosgrove says, “we are learning how nature is putting together these complex materials, and potentially that could give us ways of trying to create and design materials of equal complexity.” But, he emphasizes, his focus is not so much on making new materials as it is on building a foundation of basic science. “We’re trying to discover how nature works,” he explains. “All of our technologies come out of a basic understanding of how nature does things. Knowledge is power, and it puts you in a position where you can figure out how to tweak things to make them more useful for specific purposes. With every technology, you can go back and look at its origin, and it all comes back to basic science: Once you understand it, then you can start thinking about tinkering with it.”
Daniel Cosgrove is Professor and Holder of the Eberly Chair in Biology and director of the Center for Lignocellulose Structure and Formation at Penn State.