Rumors of the detection of gravitational waves had been circulating for months. Major news outlets were reporting that this significant news was in the making, and speculation among reputable scientists was making its rounds on Twitter and other social media outlets. Even Nature, one of the most highly respected scientific journals in the world, had published two articles in September 2015 and January 2016 that reported on the rumored gravitational waves. Excitement about this potential discovery was mounting, but the Laser Interferometer Gravitational-Wave Observatory (LIGO) was remaining mum.
Then, on February 8, LIGO, in conjunction with the National Science Foundation (NSF), announced that scientists would soon be providing an update on the search for gravitational waves. That press release led to a science media eruption—news outlets and social media users were certain they knew the outcome, and on February 11 at 10:30 a.m., the suspected, incredible news was confirmed to rejoicing scientists everywhere: gravitational waves had been detected and a new window into the Universe had been opened; the nearly imperceptible “chirp” of the gravitational wave was heard around the world. This detection of gravitational waves not only confirmed Albert Einstein’s theory of general relativity, dating to 1916, but also enabled scientists to witness the collision of two black holes.
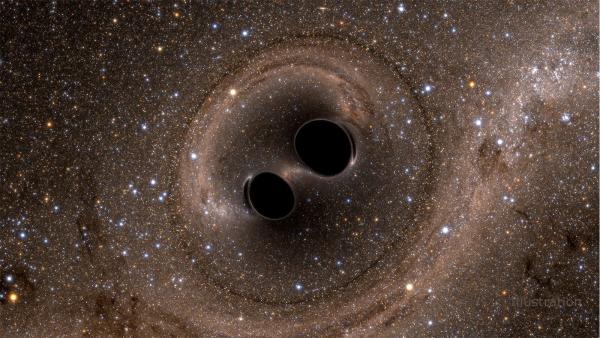
Understanding Gravitational Waves
Gravitational waves are ripples in the fabric of space-time and are a result of violent, massive events in the Universe, such as the colliding of black holes and collapsing supernovae. In this case, the gravitational wave detected in September 2015 was a result of two black holes colliding into one massive black hole nearly 1.3 billion years ago.
This remarkable news was especially exciting to the Penn State community; one of Penn State’s own physicists, Assistant Professor Chad Hanna, played a leadership role in the analysis that led to this discovery.
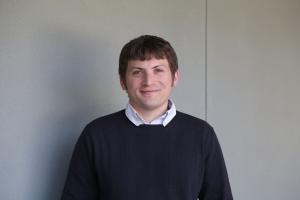
“Gravitational waves are quite literally ripples in the fabric of our Universe,” said Hanna. “This new way of discovering how the Universe functions gives us the ability to begin to understand the Universe in a way that simply has been impossible before.”
Hanna started studying gravitational waves in the summer of 2003 as an undergraduate physics student at Penn State, joined the LIGO Scientific Collaboration in 2004, and returned to Penn State as a faculty member in 2014.
“After a decade of working with LIGO, always pressing onward to try and make a discovery possible, it’s almost impossible to believe it’s happened,” Hanna said. Hanna and some of his collaborators at LIGO and Penn State have spent the majority of their careers working to achieve this groundbreaking discovery.
Hanna leads a group of Penn State researchers conducting research on gravitational-wave science. Penn State’s Institute for Gravitation and the Cosmos, of which Hanna is a member, has had an important role in developing gravitational-wave science for two decades. Researchers in the institute have made influential contributions to all theoretical aspects of gravitational wave science, including mathematical and numerical general relativity, interface with astronomy and particle astrophysics, and data analysis. The institute, directed by Abhay Ashtekar, holder of the Eberly Chair in Physics, seeks a greater understanding of the physical universe by promoting interdisciplinary efforts to develop and study fundamental theories.
“The institute’s contributions to gravitational- wave science go well beyond those by researchers currently at Penn State. Our past members now lead important groups all over the world. For over two decades, we have also trained students and postdocs and several of them have chaired key search groups and played other leadership roles in LIGO and its European partner VIRGO,” said Ashtekar.
Laying the Groundwork
The LIGO Scientific Collaboration began in 1997 as a search by American scientists to detect gravitational waves. Today, more than 1,000 scientists worldwide collaborate on the project, including Hanna, who serves as a co-chair for LIGO’s Compact Binary Coalescence research group, which determined the astrophysics behind the event that was detected.
Although the LIGO collaboration has spent the last 19 years working diligently to detect gravitational waves, it wouldn’t have been possible without the work of Professor Joseph Weber, a physicist at the University of Maryland. Weber was the first to realize it may be possible to detect gravitational waves, and in the 1960s, he invented the first resonant-mass gravitational wave detector, known as a bar detector. For the bar experiment, Weber hung an enormous bar in an insulated vacuum chamber. He compared the vibrations in the bar with the vibrations in other bars located in facilities hundreds or thousands of miles away. If the separated bars vibrated in coincidence, one could claim this as evidence for the existence of gravitational waves. While bar detectors did not have the sensitivity required for measuring gravitational waves produced by realistic astrophysical sources, this effort laid the groundwork for future detectors. Weber’s work and profound influence gave scientists around the world the ability and inspiration to later develop the advanced laser interferometer gravitational wave detectors that were used for LIGO’s gravitational waves detection in September.
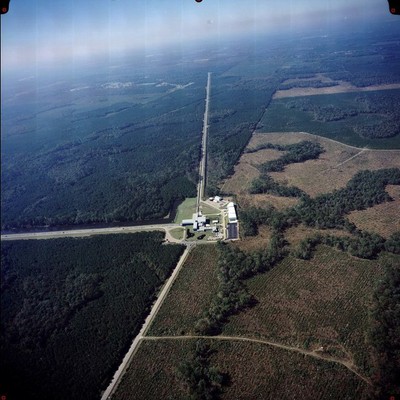
LIGO operates two gravitational-wave observatories: the LIGO Livingston Observatory in Livingston, Louisiana, and the LIGO Hanford Observatory on the Department of Energy’s Hanford site in Richland, Washington. The two observatories, which are 1,865 miles apart, are separated by this distance for a specific reason. Since the detectors are ultra-sensitive, they can detect the tiniest vibrations on Earth. If the instruments were in close proximity, there is a likelihood that they would detect the same vibrations at the same time, making it very difficult for scientists to distinguish between a vibration from an earthquake or from a gravitational wave. Having significant distance between the instruments lessens the chance that both facilities are detecting the same Earth sources at the same time. This distance allows scientists to compare data from both observatories and look for vibrations that occur at the same time, enabling them to dissect gravitational waves from Earth noise.
LIGO operates two gravitational-wave observatories: the LIGO Livingston Observatory in Livingston, Louisiana, and the LIGO Hanford Observatory on the Department of Energy’s Hanford site in Richland, Washington. The two observatories, which are 1,865 miles apart, are separated by this distance for a specific reason. Since the detectors are ultra-sensitive, they can detect the tiniest vibrations on Earth. If the instruments were in close proximity, there is a likelihood that they would detect the same vibrations at the same time, making it very difficult for scientists to distinguish between a vibration from an earthquake or from a gravitational wave. Having significant distance between the instruments lessens the chance that both facilities are detecting the same Earth sources at the same time. This distance allows scientists to compare data from both observatories and look for vibrations that occur at the same time, enabling them to dissect gravitational waves from Earth noise.
Each observatory is made up of two sections of four kilometers of evacuated pipe with lasers inside, meeting in an “L” shape. Inside this vacuum tunnel, a beam splitter divides a single entering laser beam into two beams, each traveling along one arm of the L. The beams reflect back and forth between mirrors that are suspended near each end station and near the vertex on either side of the beam splitter.
When a gravitational wave passes, the lengths of the paths that the divided laser beams take along each arm stretches the laser beam slightly, by only 1/10,000th of the diameter of a proton. This altered arm length causes each beam to travel a different distance, which indicates a gravitational wave.
Because LIGO detectors are in many respects the most sensitive instruments ever designed, in their initial proposal to NSF in the 1990s, the LIGO team had made a long-term plan in which the sensitivity was to be increased in steps. The penultimate detector was put to use in 2002 and searched for gravitational waves until 2010. As was anticipated in the NSF proposal, no gravitational waves were detected. However, the “null” result still led to some interesting bounds on astrophysical and cosmological processes. Over the next four years, LIGO scientists implemented the final improvements using sophisticated engineering and new, superior technology. A year later, Advanced LIGO was ready to detect—or rather “hear”—because the signal is in the same frequency range as the sound waves that are audible to us—cosmic explosions from the far corners of the universe.
“Hearing” the Universe
On September 14, 2015, in the middle of the night, Hanna should have gotten the most important text message of his professional life. But it was so early in Advanced LIGO’s first observing run that he didn’t even have his text message alerts set up yet. In fact, many members of the scientific collaboration thought the gravitational wave detection, called GW150914, was merely a system test rather than a real-life event.
Shortly before 2:00 p.m. that day, right before a weekly institute seminar, Hanna was caught off-guard. He received word from LIGO that no tests had been performed on the system that day, meaning that gravitational waves had actually been detected.
Hanna and his Penn State LIGO group, including postdoctoral scholars Sydney Chamberlin and Duncan Meacher, and doctoral candidate Cody Messick, arrived at the seminar stunned. (Computational scientist Alex Pace would join the group a few months later and learn about the discovery on his first day of work.) Their years of work had finally come to fruition. As in any large scientific collaboration, LIGO has a protocol that requires a long series of internal tests and detailed data analysis before major results are announced to the outside world. Therefore, as overwhelming and exciting as it was, the event was not public knowledge, so Hanna and his team had to contain the momentous news.
“The rest of our colleagues in attendance were not part of LIGO, so we couldn’t say a word. Our silence stood for months to come,” Hanna said.
On February 11, 2016, after weeks of assessing the significance of GW150914 and collaborating on scientific publications about the detection, Hanna and his colleagues at LIGO and Penn State were finally allowed to let the world know what they had learned on September 14 of the previous year: gravitational waves do exist, and the goal the LIGO scientists had been working on since its inception had finally been achieved.
How did it feel to let the world in on the news that gravitational waves had been detected?
“Relief. Vindication. Anxiety about what to work on for the next ten years?” Hanna joked. “This has been a long road for me, but it doesn’t even compare to some of my colleagues who have been working in this field for decades. I like to think, though, that I felt at least a fraction of their joy when we finally made the discovery we have all worked so hard toward.”
Finding His Niche
Hanna began studying gravitational waves as a junior in college, conducting summer research on a scholarship at Penn State. Hanna worked with Ben Owen, currently a professor of physics at Texas Tech University and adjunct professor of physics at Penn State.
After graduating with his bachelor’s degree in physics from Penn State in 2004, Hanna began graduate study at Louisiana State University, but his Penn State and LIGO connections continued. Hanna’s graduate adviser was Gabriela Gonzalez, former Penn State assistant professor of physics and current spokesperson for LIGO. Although he didn’t have a chance to work with Gonzalez before she left Penn State in 2001, her passion for her work, including LIGO, is what sold Hanna on attending Louisiana State University for his doctoral degree.
“When deciding on graduate school, I visited her group at LSU, and I confess, I didn’t think I would decide to go there before visiting. However, after meeting her I knew that she was someone with vision and passion and would not stop at anything to make sure her group would have the resources it needed to succeed. I did a full 180 and after a 26-hour visit to Baton Rouge and the LIGO Livingston Facility, I was hooked.”
When Hanna first started working with LIGO in graduate school, he was working on LIGO detector characterization. But as he worked on the project, his research took a different turn. “I soon became hooked on actually looking for signals in the data,” he said. This led him to work with the Compact Binary Coalescence group instead, one of LIGO’s largest astrophysics working groups.
The Compact Binary Coalescence group looks for signals from two compact bodies, such as neutron stars or black holes that are in a spiral motion around each other and finally merge. These sources can be from binary systems like two neutron stars, two black holes, or a combination of a neutron star and a black hole. The way the Compact Binary Coalescence group looks for these events is to notice decreases in the distance of these objects’ orbital distances, followed by an increase in orbital speed, meaning that the two objects are going to combine and become one.
Right before the coalescence happens, the gravitational waves around the event would increase in frequency until the systems combine. The entire process lasts about ten seconds, which makes picking up on these signals all the more challenging for LIGO.
Hanna’s team at Penn State leads the real-time compact binary search and has developed techniques that allow for real-time detection of gravitational waves from merging compact binary systems.
The Compact Binary Coalescence group’s work has allowed for LIGO teams to detect binary system coalescence by detecting gravitational waves—in addition to electromagnetic particles, and high-energy particles detected by other means—which is significant. As an example, in the past, the only way to detect a black hole colliding with a neutron star was to observe the powerful burst of light emitted from the neutron star. Now LIGO can detect this event also by the effect it has on space-time through gravitational waves and the presence of electromagnetic or high-energy particles near the event. In collisions between two black holes, there are no other signals. Therefore, gravitational waves provide the only means to learn about these spectacular events in which more energy is emitted during the last few seconds than are emitted by all stars in the Universe combined!
Into the Future
With this discovery, Hanna and his team at Penn State, as well as scientists around the world, are looking forward to understanding the Universe from a new perspective, where they can now listen to what they could not previously see. Having the ability to hear the Universe will allow scientists to observe the large portion of the Universe that is dark.
Hanna knows that where LIGO is right now with this research is only scratching the surface of the project’s potential. “I hope that ‘gravitational-wave observatory’ becomes as much of a household term as ‘telescope,’” he said. “The point is, over time, gravitational waves will be another way that we understand the Universe.”
Ashtekar agrees that this is only the beginning: “This first direct detection of gravitational waves is a breathtaking discovery that will stand out among the major achievements of 21st-century science because it opens the door to many discoveries that I believe will be made in the coming decade.”