To investigate potential early steps taken by the first life to develop on Earth, researchers have been studying a model of pre-life protocells comprising membraneless compartments. Now, a team of Penn State scientists have found that RNA molecules within these compartments fold better when they have naturally occurring chemical modifications. These modifications that allow for better folding in RNAs may offer a hint into how the molecules evolved from arbitrary chemical compounds to the dynamic, organized building blocks of life. The new study, published Sept. 20 by a team of Penn State scientists in the journal Science Advances, used high-throughput genetic sequencing to determine the structure of the RNAs which also has implications for the design of delivery methods for RNA-based therapeutics that rely on properly folded RNAs to function.
“RNA is similar to DNA in that it can encode genetic information in its sequence, but it also carries out many cellular functions, acting, for example, to regulate gene expression and as an enzyme that catalyzes cellular reactions,” said Phil Bevilacqua, department head of chemistry and Distinguished Professor of Chemistry and of Biochemistry and Molecular Biology in the Eberly College of Science at Penn State, and one of the leaders of the research team. “This has led to the RNA-world hypothesis, which suggests that the earliest life on Earth may have relied on RNA as both a carrier of genetic information and as a catalyst for reactions. We can never know the first steps that led to life precisely, but we hope our research can help us to narrow down what was possible on the early Earth and understand limitations in the processes that led to the origins of life.”
The so-called “primordial soup” of the oceans covering early Earth likely contained a heterogenous mixture of the chemical compounds, including those that make up RNA, that became the building blocks of life. The soup, however, would have only been an unsatisfyingly thin broth. The building blocks of life must have been incredibly dilute, Bevilacqua said, so a way of concentrating them into confined compartments, or protocells, was likely to be important for the compounds to interact with one another in the chemical reactions that eventually led to life. And the conditions in those compartments would need to be compatible with RNA folding and function.
“We are able to make membraneless compartments through liquid-liquid phase separation that mimics one way that protocells might have formed on the early Earth,” said Christine Keating, Shapiro Professor of Chemistry at Penn State and a leader of the research team. “These compartments form naturally in a mixture of oppositely charged simple polymers — in this case we are using short strings of amino acids called peptides — and we have shown previously that RNA concentrates inside of the compartments. We therefore wanted to know how sequestration in these compartments under various conditions impacted RNA’s three-dimensional structures, which are crucial for its function.”
The researchers made the compartments — called complex coacervates — using a variety of conditions. They used two different length peptides, mixtures that varied the ratio of positively and negatively charged peptides, and different concentrations of magnesium ions. In all cases, the compartments had similar physical properties and were able to sequester RNA molecules.
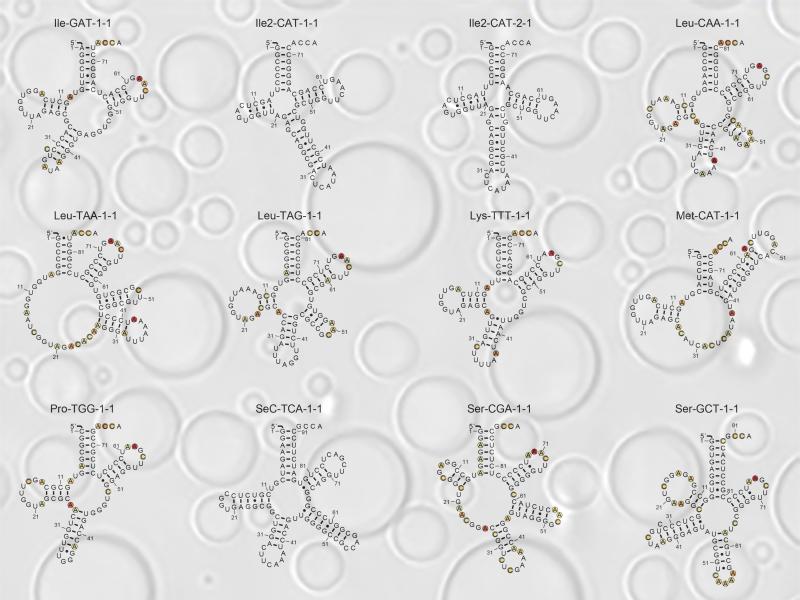
The research team then used transfer RNA (tRNA) molecules to examine RNA folding in the compartments. They chose tRNAs because they have well-studied secondary and tertiary structures, are known to be highly chemically modified in cells, and are shared across all life, suggesting that they are likely to have been present in the ancient common ancestor of life. Molecules of tRNA fold into a cloverleaf-like secondary structure and further fold into a complex three-dimensional tertiary structure. In cells, they are involved in transporting the amino acids that are used build proteins.
“We first used a single type of an unmodified tRNA molecule and determined its structure using a technique called in-line probing in the compartments that were made with different combinations of the three variables,” said McCauley Meyer, who recently earned his doctorate in biochemistry, microbiology and molecular biology at Penn State and is the first author of the paper. “The tRNA molecules folded better with higher concentrations of magnesium ions, shorter peptides, and a ratio higher in negatively charged peptides.”
In modern cells, Meyer said, chemical modifications to RNA molecules help ensure their proper folding and stability, which is crucial to their function. To test how chemical modifications to tRNA molecules impacted their folding in the membraneless compartments, the team extracted naturally modified tRNA molecules from bacterial cells and compared their folding to unmodified tRNAs in the compartments.
“We tested three of the set of conditions from the previous experiment and in all cases the modified tRNAs folded better, with fewer misfolds, than their unmodified counterparts,” Meyer said. “Here we used a high-throughput genetic sequencing technique developed in our lab, called tRNA structure-seq, that allowed us to determine the structure of several different tRNA molecules from the compartments at once.”
While researchers knew that natural modifications are important for RNA structure and stability in modern cells, Meyer said that the new research suggests they may have also been important for the early development of life on Earth.
“Using tRNAs from modern cells that have modern chemical modifications, we’ve demonstrated the principle that modifications can help with RNA folding in challenging environments inside protocells,” Bevilacqua said. “It will be for future research to establish how such modifications could have come about on the early Earth.”
The researchers also point out that the loss of RNA modification has also been implicated in human disease and that chemical modifications are important for the function of RNA therapeutics, like vaccines.
“The techniques and tools we developed in this research could help us to understand how modifications to RNA impact human health and aid in the development of new RNA drugs,” Meyer said.
In addition to Bevilacqua, Keating, and Meyer, the research team at Penn State includes Ryota Yamagami, a postdoctoral researcher in the Bevilacqua lab at the time of the research who is now an assistant professor at Ehime University in Japan, and Saehyun Choi, a graduate student in the Keating lab at the time of the research who is now a postdoctoral researcher at the University of California, Berkeley. Members of the research team are part of the Center for RNA Molecular Biology and the Astrobiology Research Center at Penn State.
The NASA Exobiology program and the U.S. National Institutes of Health funded this research.
Future Investigators in NASA Earth and Space Science and Technology (FINESST) and an Overseas Research Fellowship from the Japan Society for the Promotion of Science provided additional support.